Air Resistance: Definition, Type, Difference
Jim Goodrich • • Reading time: 10 min
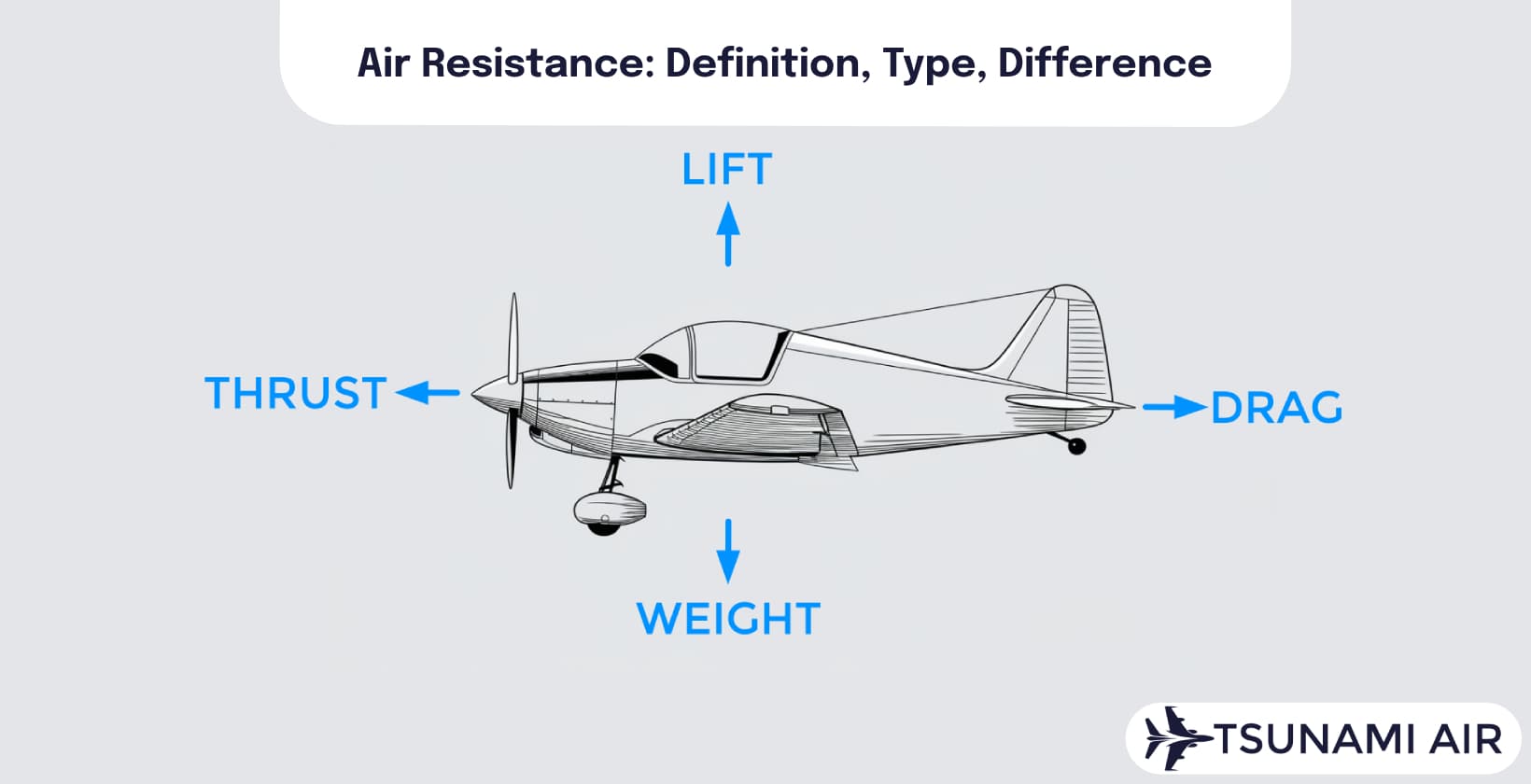
Air resistance is the force exerted on an object as it moves through air. Air resistance has types and characteristics that define its behavior. Air resistance impacts the motion of objects in different ways, influencing their speed and trajectory. Air resistance plays a part in many fields, including aerodynamics and physics. Understand the definition and types of air resistance to grasp its importance in phenomena and scientific applications.
Air resistance is a type of force opposing object motion through air. The magnitude of air resistance depends on factors like velocity, object properties, and air characteristics. Air resistance follows a square relationship with speed, meaning higher speeds result in greater resistance.
Air resistance differs from drag in its application to air as a medium. Drag is a term for resistive forces in any fluid, while air resistance refers to forces opposing motion through air. Drag encompasses components, including lift-induced, parasitic, and wave drag. Drag force magnitude increases with velocity squared and is measured using the formula F_D = 1/2 ρv^2 C_D A, where ρ represents air density, v is velocity, C_D is the drag coefficient, and A is the cross-sectional area. Drag coefficient characterizes shape effects on drag force and influences streamlining and drag reduction efforts.
What is the definition of air resistance?
The definition of air resistance is a force that opposes the motion of objects through air. Air resistance results from collisions between objects and air molecules, creating friction that slows objects down. Air resistance acts opposite to the direction of motion and increases with speed.
Air resistance opposes motion, acting as a vector in the opposite direction of an object's movement. Air acts as a fluid medium with specific density and mass concentration, influencing resistance strength.
Velocity affects air resistance. Higher speeds result in greater resistance, following a square relationship. Object properties play a part in determining air resistance. Shape impacts drag, with streamlined forms experiencing less resistance than irregular shapes. Surface area exposed to airflow increases resistance proportionally. Drag coefficient represents a dimensionless factor dependent on object shape, used in calculations to determine resistance.
Physics of air resistance involves friction and surface interactions between air molecules and moving objects. Aerodynamic forces contribute to the resistance experienced by objects in motion. Flow dependency affects resistance, with turbulent flow creating more drag than laminar flow. Air resistance manifests in different forms, including form drag and skin friction drag. Form drag results from pressure differences around an object, while skin friction drag occurs due to direct contact between air molecules and object surfaces.
What is the equation for air resistance?
The equation for air resistance is FD = 1/2 ρ CD A v^2, where FD represents the drag force, ρ is air density, CD is the drag coefficient, A is the reference area, and v is velocity. Drag force calculation requires values for ρ (1.225 kg/m³ (0.0765 lb/ft³)), CD, A, and v. Air density measures 1.225 kg/m³ (0.0765 lb/ft³) at sea level. Drag coefficients vary for different shapes, with a sphere having a value of 0.47 and a flat plate 1.28. Velocity magnitude affects air resistance due to its squared relationship, with doubling speed increasing drag force by a factor of four.
What type of force is air resistance?
Air resistance is a type of force that opposes motion of objects through air. Air resistance is a kind of friction force occurring when objects move through fluids. Air resistance increases with velocity and cross-sectional area, affecting aviation, sports projectiles, and automotive design.
Air resistance is classified as a drag force. Drag force depends on velocity and increases with the square of velocity. The drag force calculation uses the formula F_D = 1/2 ρv^2 C_D A, where variables represent drag force, air density, velocity, drag coefficient, and cross-sectional area. Drag constitutes friction and acts against object motion, opposing the direction of movement and reducing object speed over time.
Air resistance functions as a friction force. Friction acts as a contact force between surfaces, resisting motion or attempted motion. Friction causes energy loss by converting mechanical energy to heat. Air resistance exhibits characteristics of a non-conservative force. Non-conservative forces have a path-dependent nature and cause energy dissipation. These forces convert mechanical energy to other forms and generate non-recoverable heat.
Air resistance operates as a dissipative force. Dissipative forces convert mechanical energy to thermal energy and disperse it to the surrounding. The work performed by dissipative forces is non-recoverable, preventing the reuse of energy for mechanical work. Air resistance causes a loss of mechanical energy in moving objects, contributing to the system's energy loss.
How does air resistance affect falling objects?
Air resistance affects falling objects by counteracting gravity. Air resistance exerts force against gravity, decreasing acceleration. Influence depends on the object's speed and cross-sectional area. Air resistance increases with speed, balancing gravity at terminal velocity. Objects fall due to greater gravity force.
Air resistance on falling objects is influenced by mass, shape, and surface area. Heavier objects experience greater gravitational force, allowing them to accelerate before air resistance becomes considerable. Aerodynamic shapes reduce drag, while irregular shapes increase it. Objects with larger surface areas encounter more air resistance due to increased collisions with air molecules.
Air density affects the magnitude of air resistance on falling objects. Denser air results in more air resistance than thinner air. The drag coefficient value depends on the object's shape and orientation. A sphere has a drag coefficient of 0.47, while a flat plate has a higher value depending on its orientation.
Gravity force and air resistance force interact during an object's fall. Gravity acceleration causes objects to fall at 9.81 m/s² (32.2 ft/s²) on Earth, assuming no air resistance. Air resistance force increases with velocity, counteracting gravity. The drag force is calculated using the formula F_D = 1/2CρAv², where C is the drag coefficient, ρ is air density, A is the cross-sectional area, and v is the velocity. Acceleration changes throughout the fall as air resistance increases.
Terminal velocity occurs when air resistance equals gravity force. Objects reach a steady state with speed at terminal velocity. Factors affecting terminal velocity include object mass, shape, surface area, and air properties. Lighter objects or those with large surface areas reach terminal velocity faster due to greater air resistance relative to their weight. Terminal velocity represents the maximum speed an object can achieve during free fall in air.
What is the difference between air resistance and drag?
The difference between air resistance and drag is that air resistance refers to forces opposing motion through air, while drag is a broader term for resistive forces in any fluid. Air resistance is a type of drag that occurs in air.
The difference between air resistance and drag is explained in the table below.
Aspect | Air Resistance | Drag |
Definition | A force that opposes an object's motion through air. | A force that opposes an object's motion through any fluid (liquid or gas). |
Mechanism | Occurs due to collisions between object surfaces and air molecules. | Results from factors including form drag, skin friction drag, lift-induced drag, and wave drag. |
Velocity Dependence | Force increases with the square of the object's speed (v^2). | Magnitude depends on velocity (v or v^2), fluid density (ρ), and object characteristics. |
Measurement | Usually assessed via wind tunnels or computational simulations. | Measured using wind tunnels, computational simulations, and by determining drag coefficients (C_d). |
Drag Force Definition | Specific to air; considered a type of drag through air. | Force that opposes motion through fluids, given by F_d = ½ ρ v^2 C_d A. |
Magnitude Dependence | Increases with speed squared, similar to general drag. | Increases with the square of velocity for non-viscous fluids. |
Drag Coefficient | Related to air-specific forces and object interaction with air (e.g., C_d = 0.45 for a sphere). | Dimensionless number characterizing shape effects on drag (e.g., C_d = 0.05 for an airfoil). |
Components | N/A | Includes form drag, skin friction drag, lift-induced drag, and wave drag. |
Form Drag | N/A | Arises from pressure differences around an object; affected by body shape. |
Skin Friction Drag | N/A | Results from fluid viscosity and surface roughness. |
Drag force measurement determines drag coefficient. Drag coefficient characterizes shape effects on drag force. Drag coefficient is a dimensionless number that quantifies drag relative to size and speed. Drag coefficient shape factor influences streamlining and drag reduction.
Form drag arises from pressure distribution around objects. Pressure distribution affects drag between an object’s front and rear. Body shape impacts streamlining and drag reduction. Skin friction results from fluid-surface friction. Skin friction drag results from viscosity related to fluid properties and roughness.
What are some examples of air resistance force?
Examples of air resistance force include cyclists experiencing resistance while riding, parachutes utilizing air resistance to slow descent, and winds creating resistance during storms. Feathers fall due to air resistance, while aircraft designs minimize it for efficiency.
Examples of air resistance force are provided in the list below.
- Cyclists experiencing air resistance force: Cyclists face air resistance due to their frontal area, and aerodynamic designs aim to reduce this resistance.
- Parachutes maximizing air resistance force: Parachutes utilize large surface areas and high drag coefficients to slow down descent.
- Airplanes minimizing air resistance force: Airplanes use streamlined designs to reduce drag and upgrade efficiency.
- Paper planes minimizing air resistance force: Paper planes with lightweight structures and aerodynamic shapes reduce air resistance.
- Hand movements demonstrating air resistance force: Different hand orientations and wind speeds affect air resistance.
- Rockets facing air resistance force: Rockets reduce air resistance during ascent with aerodynamic profiles and pointed noses.
- Skydivers controlling air resistance force: Skydivers adjust body postures to manage air resistance during freefall.
- Falling feathers demonstrating air resistance force: Feathers experience air resistance due to their shape and mass.
Cyclists experience air resistance due to their frontal area. A cyclist's frontal area of 0.5 square meters (5.38 square feet) creates drag. Aerodynamic designs reduce this resistance. Streamlined helmets decrease turbulence by up to 10%. Handlebars lower drag by 15%.
Parachutes maximize air resistance with their large surface area. A parachute has a surface area of 30 square meters (322.92 square feet). Parachutes utilize a drag coefficient of around 1.75. This drag coefficient ensures a stable descent rate of 5 meters per second (16.4 feet per second).
Paper planes leverage their lightweight structure and aerodynamic shape to minimize air resistance. A well-designed paper plane weighs 10 grams (0.022 pounds). Pointed fronts reduce air resistance by 20%. Curved wings decrease drag by 15%.
Hand movements through air demonstrate levels of resistance. A flat hand orientation increases drag by 50% compared to an edge-on orientation. Wind velocity impacts air resistance. Wind speeds of 30 km/h (18.64 mph) double the force of air resistance compared to calm conditions. Turbulent winds create unpredictable resistance patterns. Gusts of 50 km/h (31 mph) increase air resistance by up to 300%.
Airplanes employ streamlined designs to reduce air resistance. Wings minimize drag while maximizing lift. Airplanes achieve drag coefficients between 0.02 and 0.05. Rockets face air resistance at high velocities. Aerodynamic profiles reduce air resistance during ascent. Pointed noses decrease drag by 30%. Smooth bodies reduce air resistance by 20%. Fin designs balance stability with drag reduction.
Skydivers control air resistance through body posture adjustments. A spread-eagle position increases drag by 70%. Streamlined positions reduce drag by 50%. Larger cross-sectional areas increase drag for slowing down. A head-down position reduces drag by 40% compared to a belly-to-earth position.
Falling feathers demonstrate susceptibility to air resistance. Feathers have a mass of 0.1 grams (0.00022 pounds). Irregular shapes create drag coefficients of up to 1.5. Feathers descend 5 times slower than streamlined objects of similar mass due to their shape.